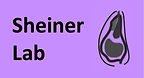

SheinerLab
Why is it important to study divergent organisms?
Exploring new biological systems has often lead to the discovery of new biological principles and new biotechnological tools. At a time of growing understanding of the true extent of eukaryotic diversity, investigation of the biology of organisms from across the eukaryotic tree is urgently required. This task is currently largely unfulfilled, including with regards to the biology of endosymbiotic organelles (e.g. mitochondria and plastids). Much of the available knowledge of the biology of these organelles comes from work in Ophistokonta (e.g. yeast, worms, fruit flies, rodents and humans) and Archaeplastida (e.g. plants, green and red algae), leaving the larger part of eukaryotic lineages understudied.
What are we studying?
We study Toxoplasma gondii first as an example of a divergent eukaryote, but also as a model for the phylum Apicomplexa, which includes parasites of human and veterinary medical importance like Plasmodium spp that cause malaria (we also study Plasmodium itself sometimes). By exploring Toxoplasma biology we expand the repertoire of biological systems that we understand well and discover new biological principles. While doing so we also pinpoint new parasite-specific structures and feature that are potential targets for intervention.
Our lab is focused on divergent pathways in the function and biogenesis pathways of the T. gondii organelles of endosymbiotic origin – primarily the mitochondrion, and with some work on the apicoplast too.
Mitochondria and mitochondria-like organelles are essential for most eukaryotes including apicomplexan parasites. The apicomplexan mitochondrion shows several divergent features compared to well-studied systems such as plants, fungi and metazoans: these parasites have a single large mitochondrion per cell that only divides simultaneously with cytokinesis (unlike mammalian mitochondria that may change their numbers independently of the cell cycle); Apicomplexans have the smallest known mitochondrial genome encoding only three open reading frames, one of which is the target of the antimalarial Atovaquone. The nimimal genome further includes a highly fragmented rRNA and other data suggest the existence of a highly divergent mitochondrial-ribosome in apicomplexan, for which we started accomulating support and are exploring the corresponding mechanism. Sequence analysis and a series of indirect studies also suggest divergent features of major components of the mitochondrial electron transport chain and ATP synthase, which we validated with proteomics, genetic and structural studies. Evidence further point to divergence in pathways such as mitochondrial protein and tRNA import. Finally, the mitochondrion engages in exchange with other organelles via membrane contact sites for which we are exploring the tethering and functions. In summary, our lab studied the mechanistic details of some of those pathways, where knowledge of the molecular players, their structure and their mode of action may inform new drug design.
The apicomplexa ancestor engaged in secondary endosymbiosis, thus acquiring a plastid of red algal origin, with a complex four-membrane architecture. Apicomplexan then have gone through further “life-changing” events, losing the ability to perform photosynthesis and turning to parasitism. While no longer photosynthetic the apicoplast hosts essential metabolic pathways. Both metabolic and housekeeping pathways hosted in the apicoplast stroma are known targets for anti-apicomplexan drugs. However, less attention was focused on the pathways hosted in the peripheral compartments. We explore what proteins and pathways are hosted in these compartments and characterize their roles and molecular mechanisms.

Mitochondria of two Toxoplasma parasites. Outer membrane - green; lumen - magenta.
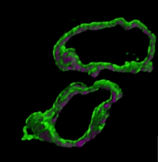
Apicoplasts (and nuclei) of two Toxoplasma parasites. DNA - blue; Outer apicoplast membrane - green; lumen - magenta.
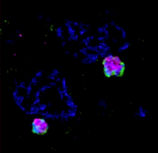
Recent Highlights
The apicomplexan mitochondrial ATP synthase forms novel higher order assemblies through hexamerization. These hexamers further form pentagonal pyramids at the tip of the mitochondrial cristae. Hexamerization is mediated by new ATP synthase components that were not found in ATP synthase of previously studied organisms. When we delet one of those new components, hexamers are no longer formed resulting in a defect in the parasite unique cristae shape.
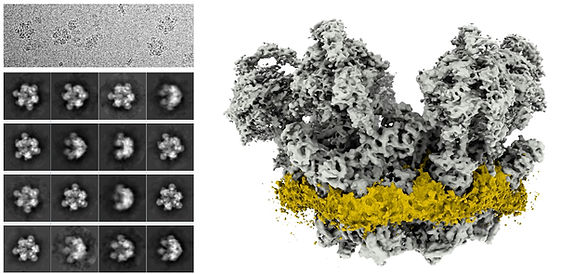


The apicomplexan mitochondrial electron transport chain (mETC) is a know target for anti-apicomplexan drugs and there is numerous evidence suggesting it is divergent from the mETC of human, yet the composition of all the complexes composing the mETC in these parasites was not known. Using complexome profiling we identified many (and potentially all) the subunits of there complexes, while discovering novel, parasite specific components.
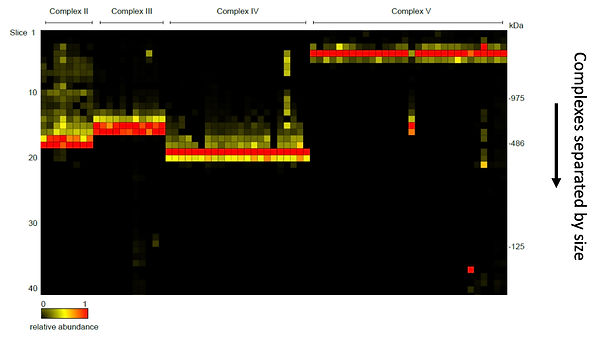
Malaria parasites produce the antioxidant Lipoic Acid (LA) in a their apicoplast. We showed that when they cannot make LA, their chemical and metabolic balance changes, and the cannot develop into their infectious form in the mosquito vector.
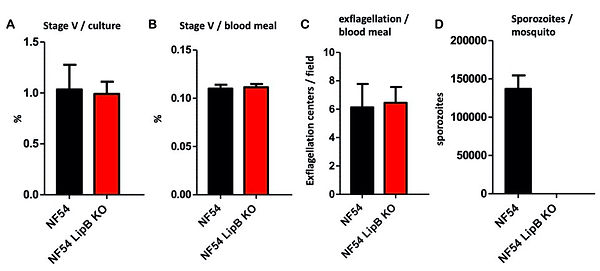
Mitochondrial translation is divergent in apicomplexan compared to human but it is understudied. In our 2019 Mol Micro paper we generated tools allowing us to study this process: we detected mito-ribosome complexes for the first time and we established a new assay to follow (indirectly but specifically) mitochondrial translation in Toxoplasma

(A) A scheme describing the rational of our assay. Nuclear (grey) encoded proteins translated by the cytosolic ribosome (green) compose complex V, while mitochondrial (yellow) encoded proteins translated by the mitochondrial ribosome (blue) are necessary to assemble complex IV. Depletion of TgmS35 (red) results in reduced activity of complex IV but not V.
(B) The assay comparing complex IV activity to complex V activity under down regulation of TgmS35 or in wild type.
An import pore like that found in plant chloroplasts is essential for Toxoplasma and the malaria causing Plasmodium parasites
Toxoplasma Toc75 is involved in apicoplast protein import
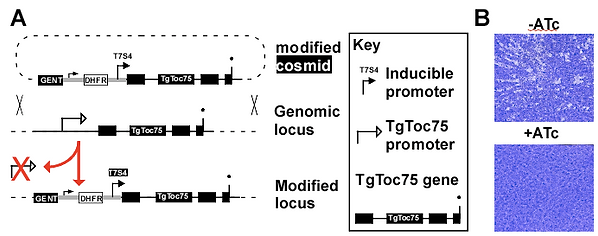
A. Strategy for genetic manipulation resulting in Toc75 inducible knock-down.
B. Growth assay showing that Toc75 is essential for parasite growth
The single mitochondrion of Toxoplasma gondii seem to form close association with its peripheral organelles. The mitochondrion then steps away from the periphery when the parasite move from one host-cell to another.
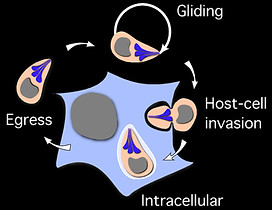